
Locked

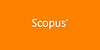

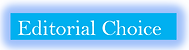
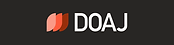
Tuesday, April 2, 2024 at 7:15:00 AM UTC
Request Open
Non-Hermitian unidirectional routing of photonic qubits
Unlock Only
Changeover the Schrödinger Equation
This option will drive you towards only the selected publication. If you want to save money then choose the full access plan from the right side.
Unlock all
Get access to entire database
This option will unlock the entire database of us to you without any limitations for a specific time period.
This offer is limited to 100000 clients if you make delay further, the offer slots will be booked soon. Afterwards, the prices will be 50% hiked.
Newsletters
Abstract
Efficient and tunable qubit unidirectional routers and spin-wave diodes play an important role in both classical and quantum information processing domains. Here, we reveal that multi-level neutral cold atoms can mediate both dissipative and coherent couplings. Interestingly, we investigate and practically implement this paradigm in experiments, successfully synthesizing a system with dual functionality as both a photonic qubit unidirectional router and a spin-wave diode. By manipulating the helicity of the field, we can effectively balance the coherence coupling and dissipative channel, thereby ensuring the unidirectional transfer of photonic qubits. The qubit fidelity exceeds 97.49 ± 0.39%, and the isolation ratio achieves 16.8 ± 0.11 dB while the insertion loss is lower than 0.36 dB. Furthermore, we show that the spin-wave diode can effectively achieve unidirectional information transfer by appropriately setting the coherent coupling parameters. Our work not only provides new ideas for the design of extensive components in quantum network, but also opens up new possibilities for non-Hermitian quantum physics, complex quantum networks, and unidirectional quantum information transfer.
Introduction
In contemporary communication and information technology, the concept of unidirectional routing for information carriers has garnered substantial attention. This concept is extensively employed in the transmission of various signals, including acoustic waves [1–3], radio frequencies [4, 5], and quantum signals [6]. Among them, gyrators serve an indispensable role as key components in facilitating efficient and orderly information exchange between different nodes [4, 7]; dual-port isolators effectively suppress reverse noise [4, 6, 8, 9]; while unidirectional amplifiers focus on the directional amplification of weak signals [10, 11]. In linear systems, the achievement of unidirectional responses hinges on the disruption of time-reversal symmetry through the application of real or synthetic magnetic fields. However, the practicality of these traditional unidirectional devices is hampered by their biased magnetic fields. In recent years, promising physical mechanisms have emerged to overcome the aforementioned limitations, including nonlinear optics [12–14], optomechanics [15, 16], atomic gases [17–21], quantum dots [22], and metamaterials [23]. The Unidirectional router and spin-wave diode simplify the intricate nature of photonic networks [8, 24], augment communication channel capacities [25, 26], and becomes valuable resources in quantum sensing [27]. Such a device promotes the development of more efficient and adaptable quantum information platforms [6, 28]. It stimulated numerous recent studies on nonreciprocal couplings and chiral magnons transfer, such as quantum transistors and transducers [29–31], quantum diodes [32–34], unidirectional amplifiers [35–38], and spin-wave diode [6, 28, 31, 39, 40]. However, as the quantum nodes increase, the cumulative effect of insertion loss and quantum coherence loss leads to a significant increase in
Reference
[1] T. Devaux, A. Cebrecos, O. Richoux, V. Pagneux, and V. Tournat, Acoustic radiation pressure for nonreciprocal transmission and switch effects, Nature Communications 10, 3292 (2019).
[2] Q. Wang, Z. Zhou, D. Liu, H. Ding, M. Gu, and Y. Li, Acoustic topological beam nonreciprocity via the rotational Doppler effect, Science Advances 8, eabq4451 (2022).
[3] L. Hackett, M. Miller, S. Weatherred, S. Arterburn, M. J. Storey, G. Peake, D. Dominguez, P. S. Finnegan, T. A. Friedmann, and M. Eichenfield, Non-reciprocal acoustoelectric microwave amplifiers with net gain and low noise in continuous operation, Nature Electronics 6, 76 (2023).
[4] A. Nagulu, N. Reiskarimian, and H. Krishnaswamy, Nonreciprocal electronics based on temporal modulation, Nature Electronics 3, 241 (2020).
[5] L. Shao, D. Zhu, M. Colangelo, D. Lee, N. Sinclair, Y. Hu, P. T. Rakich, K. Lai, K. K. Berggren, and M. Lonˇcar, Electrical control of surface acoustic waves, Nature Electronics 5, 348 (2022).
[6] P. Lodahl, S. Mahmoodian, S. Stobbe, A. Rauschenbeutel, P. Schneeweiss, J. Volz, H. Pichler, and P. Zoller, Chiral quantum optics, Nature 541, 473 (2017).
[7] G. Viola and D. P. DiVincenzo, Hall effect gyrators and 6 circulators, Physical Review X 4, 021019 (2014).
[8] D. Jalas, A. Petrov, M. Eich, W. Freude, S. Fan, Z. Yu, R. Baets, M. Popovi´c, A. Melloni, J. D. Joannopoulos, M. Vanwolleghem, C. R. Doerr, and H. Renner, What is and what is not an optical isolator, Nature Photonics 7, 579 (2013).
[9] M. Scheucher, A. Hilico, E. Will, J. Volz, and A. Rauschenbeutel, Quantum optical circulator controlled by a single chirally coupled atom, Science 354, 1577 (2016).
[10] C. C. Wanjura, M. Brunelli, and A. Nunnenkamp, Topological framework for directional amplification in drivendissipative cavity arrays, Nature Communications 11, 3149 (2020).
[11] Z. Shen, Y.-L. Zhang, Y. Chen, F.-W. Sun, X.-B. Zou, G.-C. Guo, C.-L. Zou, and C.-H. Dong, Reconfigurable optomechanical circulator and directional amplifier, Nature Communications 9, 1797 (2018).
[12] L. Fan, J. Wang, L. T. Varghese, H. Shen, B. Niu, Y. Xuan, A. M. Weiner, and M. Qi, An all-silicon passive optical diode, Science 335, 447 (2012).
[13] Q.-T. Cao, H. Wang, C.-H. Dong, H. Jing, R.-S. Liu, X. Chen, L. Ge, Q. Gong, and Y.-F. Xiao, Experimental demonstration of spontaneous chirality in a nonlinear microresonator, Physical Review Letters 118, 033901 (2017).
[14] K. Xia, F. Nori, and M. Xiao, Cavity-Free Optical Isolators and Circulators Using a Chiral Cross-Kerr Nonlinearity, Physical Review Letters 121, 203602 (2018).
[15] S. Manipatruni, J. T. Robinson, and M. Lipson, Optical nonreciprocity in optomechanical structures, Physical Review Letters 102, 213903 (2009).
[16] Z. Shen, Y.-L. Zhang, Y. Chen, C.-L. Zou, Y.-F. Xiao, X.-B. Zou, F.-W. Sun, G.-C. Guo, and C.-H. Dong, Experimental realization of optomechanically induced nonreciprocity, Nature Photonics 10, 657 (2016). [17] E.-Z. Li, D.-S. Ding, Y.-C. Yu, M.-X. Dong, L. Zeng, W.-H. Zhang, Y.-H. Ye, H.-Z. Wu, Z.-H. Zhu, W. Gao, G.-C. Guo, and B.-S. Shi, Experimental demonstration of cavity-free optical isolators and optical circulators, Physical Review Research 2, 033517 (2020).
[18] M.-X. Dong, K.-Y. Xia, W.-H. Zhang, Y.-C. Yu, Y.-H. Ye, E.-Z. Li, L. Zeng, D.-S. Ding, B.-S. Shi, G.-C. Guo, and F. Nori, All-optical reversible single-photon isolation at room temperature, Science Advances 7, eabe8924 (2021).
[19] S. Zhang, Y. Hu, G. Lin, Y. Niu, K. Xia, J. Gong, and S. Gong, Thermal-motion-induced non-reciprocal quantum optical system, Nature Photonics 12, 744 (2018).
[20] X.-X. Hu, Z.-B. Wang, P. Zhang, G.-J. Chen, Y.-L. Zhang, G. Li, X.-B. Zou, T. Zhang, H. X. Tang, C.-H. Dong, G.-C. Guo, and C.-L. Zou, Noiseless photonic nonreciprocity via optically-induced magnetization, Nature Communications 12, 2389 (2021).
[21] S. Pucher, C. Liedl, S. Jin, A. Rauschenbeutel, and P. Schneeweiss, Atomic spin-controlled non-reciprocal Raman amplification of fibre-guided light, Nature Photonics 16, 380 (2022).
[22] N. O. Antoniadis, N. Tomm, T. Jakubczyk, R. Schott, S. R. Valentin, A. D. Wieck, A. Ludwig, R. J. Warburton, and A. Javadi, A chiral one-dimensional atom using a quantum dot in an open microcavity, npj Quantum Information 8, 27 (2022).
[23] P. P. Iyer, R. A. DeCrescent, Y. Mohtashami, G. Lheureux, N. A. Butakov, A. Alhassan, C. Weisbuch, S. Nakamura, S. P. DenBaars, and J. A. Schuller, Unidirectional luminescence from InGaN/GaN quantum-well metasurfaces, Nature Photonics 14, 543 (2020).
24] A. Metelmann and H. E. T¨ureci, Nonreciprocal signal routing in an active quantum network, Physical Review A 97, 043833 (2018).
[25] D. A. Miller, Are optical transistors the logical next step?, Nature Photonics 4, 3 (2010).
[26] E. Verhagen and A. Al`u, Optomechanical nonreciprocity, Nature Physics 13, 922 (2017).
[27] H.-K. Lau and A. A. Clerk, Fundamental limits and nonreciprocal approaches in non-Hermitian quantum sensing, Nature Communications 9, 4320 (2018).
[28] H. J. Kimble, The quantum internet, Nature 453, 1023 (2008).
[29] H. Gorniaczyk, C. Tresp, J. Schmidt, H. Fedder, and S. Hofferberth, Single-photon transistor mediated by interstate Rydberg interactions, Physical Review Letters 113, 053601 (2014).
[30] Z. Wang, Z. Bao, Y. Li, Y. Wu, W. Cai, W. Wang, X. Han, J. Wang, Y. Song, L. Sun, H. Zhang, and L.- M. Duan, An ultra-high gain single-photon transistor in the microwave regime, Nature Communications 13, 6104 (2022).
[31] K. Stannigel, P. Rabl, A. S. Sørensen, P. Zoller, and M. D. Lukin, Optomechanical transducers for longdistance quantum communication, Physical Review Letters 105, 220501 (2010)
[32] A. RosarioHamann, C. M¨uller, M. Jerger, M. Zanner, J. Combes, M. Pletyukhov, M. Weides, T. M. Stace, and A. Fedorov, Nonreciprocity realized with quantum nonlinearity, Physical Review Letters 121, 123601 (2018).
[33] C. M¨uller, J. Combes, A. R. Hamann, A. Fedorov, and T. M. Stace, Nonreciprocal atomic scattering: A saturable, quantum Yagi-Uda antenna, Physical Review A 96, 053817 (2017).
[34] S. Barzanjeh, M. Aquilina, and A. Xuereb, Manipulating the flow of thermal noise in quantum devices, Physical Review Letters 120, 060601 (2018).
[35] A. Metelmann and A. A. Clerk, Nonreciprocal photon transmission and amplification via reservoir engineering, Physical Review X 5, 021025 (2015).
[36] F. Lecocq, L. Ranzani, G. A. Peterson, K. Cicak, R. W. Simmonds, J. D. Teufel, and J. Aumentado, Nonreciprocal microwave signal processing with a fieldprogrammable Josephson amplifier, Physical Review Applied 7, 024028 (2017).
[37] G. A. Peterson, F. Lecocq, K. Cicak, R. W. Simmonds, J. Aumentado, and J. D. Teufel, Demonstration of efficient nonreciprocity in a microwave optomechanical circuit, Physical Review X 7, 031001 (2017). [38] D. Malz, L. D. T´oth, N. R. Bernier, A. K. Feofanov, T. J. Kippenberg, and A. Nunnenkamp, Quantum-limited directional amplifiers with optomechanics, Physical Review Letters 120, 023601 (2018).
[39] J. I. Cirac, P. Zoller, H. J. Kimble, and H. Mabuchi, Quantum state transfer and entanglement distribution among distant nodes in a quantum network, Physical Review Letters 78, 3221 (1997).
[40] J. Lan, W. Yu, R. Wu, J. Xiao, et al., Spin-wave diode, Physical Review X 5, 041049 (2015). [41] See Supplemental Material for more details, [42] R. Wen, C.-L. Zou, X. Zhu, P. Chen, Z. Y. Ou, 7 J. F. Chen, and W. Zhang, Non-Hermitian magnonphoton interference in an atomic ensemble, Physical Review Letters 122, 253602 (2019). [43] J. Simon, H. Tanji, S. Ghosh, and V. Vuleti´c, Singlephoton bus connecting spin-wave quantum memories, Nature Physics 3, 765 (2007). [44] G.-S. Ye, B. Xu, Y. Chang, S. Shi, T. Shi, and L. Li, A photonic entanglement filter with Rydberg atoms, Nature Photonics 17, 538 (2023). [45] D.-S. Ding, W. Zhang, Z.-Y. Zhou, S. Shi, B.-S. Shi, and G.-C. Guo, Raman quantum memory of photonic polarized entanglement, Nature Photonics 9, 332–338 (2015). [46] Y.-W. Cho and Y.-H. Kim, Atomic vapor quantum memory for a photonic polarization qubit, Optics Express 18, 25786 (2010). [47] C. Gonzalez-Ballestero, A. Gonzalez-Tudela, F. J. Garcia-Vidal, and E. Moreno, Chiral route to spontaneous entanglement generation, Physical Review B 92, 155304 (2015). [48] A. V. Gorshkov, A. Andr´e, M. Fleischhauer, A. S. Sørensen, and M. D. Lukin, Universal approach to optimal photon storage in atomic media, Physical Review Letters 98, 123601 (2007). [49] L.-M. Duan, M. D. Lukin, J. I. Cirac, and P. Zoller, Longdistance quantum communication with atomic ensembles and linear optics, Nature 414, 413 (2001). [50] Y.-C. Chen, C.-W. Lin, and I. A. Yu, Roles of degenerate Zeeman levels in electromagnetically induced transparency, Physical Review A 61, 053805 (2000). [51] B. Wang, S. Li, J. Ma, H. Wang, K. C. Peng, and M. Xiao, Controlling the polarization rotation of an optical field via asymmetry in electromagnetically induced transparency, Physical Review A 73, 051801 (2006). [52] F. Alpeggiani, K. Y. Bliokh, F. Nori, and L. Kuipers, Electromagnetic helicity in complex media, Physical Review Letters 120, 243605 (2018). [53] K. Y. Bliokh, Y. S. Kivshar, and F. Nori, Magnetoelectric effects in local light-matter interactions, Physical Review Letters 113, 033601 (2014). [54] T. Van Mechelen and Z. Jacob, Universal spinmomentum locking of evanescent waves, Optica 3, 118 (2016). [55] Z.-Q. Yang, Z.-K. Shao, H.-Z. Chen, X.-R. Mao, and R.- M. Ma, Spin-momentum-locked edge mode for topological vortex lasing, Physical Review Letters 125, 013903 (2020). [56] V. Bali´c, D. A. Braje, P. Kolchin, G. Y. Yin, and S. E. Harris, Generation of paired photons with controllable waveforms, Physical Review Letters 94, 183601 (2005). [57] S. Du, J. Wen, and M. H. Rubin, Narrowband biphoton generation near atomic resonance, JOSA B 25, C98 (2008). [58] M. Fleischhauer, A. Imamoglu, and J. P. Marangos, Electromagnetically induced transparency: Optics in coherent media, Reviews of Modern Physics 77, 633 (2005). [59] D. F. V. James, P. G. Kwiat, W. J. Munro, and A. G. White, Measurement of qubits, Physical Review A 64, 052312 (2001). [60] K. Koshino, S. Ishizaka, and Y. Nakamura, Deterministic photon-photon SWAP gate using a Λ system, Physical Review A 82, 010301 (2010). [61] L.-M. Duan and H. J. Kimble, Scalable photonic quantum computation through cavity-assisted interactions, Physical Review Letters 92, 127902 (2004). [62] B. Pingault, J. N. Becker, C. H. H. Schulte, C. Arend, C. Hepp, T. Godde, A. I. Tartakovskii, M. Markham, C. Becher, and M. Atat¨ure, All-Optical Formation of Coherent Dark States of Silicon-Vacancy Spins in Diamond, Physical Review Letters 113, 263601 (2014). [63] L. J. Rogers, K. D. Jahnke, M. H. Metsch, A. Sipahigil, J. M. Binder, T. Teraji, H. Sumiya, J. Isoya, M. D. Lukin, P. Hemmer, and F. Jelezko, All-Optical Initialization, Readout, and Coherent Preparation of Single Silicon-Vacancy Spins in Diamond, Physical Review Letters 113, 263602 (2014). [64] K. Xia, F. Jelezko, and J. Twamley, Quantum routing of single optical photons with a superconducting flux qubit, Physical Review A 97, 052315 (2018). [65] M.-M. Cao, K. Li, W.-D. Zhao, W.-X. Guo, B.-X. Qi, X.- Y. Chang, Z.-C. Zhou, Y. Xu, and L.-M. Duan, Probing Complex-Energy Topology via Non-Hermitian Absorption Spectroscopy in a Trapped Ion Simulator, Physical Review Letters 130, 163001 (2023). [66] H. Cao and J. Wiersig, Dielectric microcavities: Model systems for wave chaos and non-Hermitian physics, Reviews of Modern Physics 87, 61 (2015). [67] R. El-Ganainy, K. G. Makris, M. Khajavikhan, Z. H. Musslimani, S. Rotter, and D. N. Christodoulides, NonHermitian physics and PT symmetry, Nature Physics 14, 11 (2018). [68] S. Bernon, H. Hattermann, D. Bothner, M. Knufinke, P. Weiss, F. Jessen, D. Cano, M. Kemmler, R. Kleiner, D. Koelle, et al., Manipulation and coherence of ultracold atoms on a superconducting atom chip, Nature Communications 4, 2380 (2013). [69] L. Zhu, X. Liu, B. Sain, M. Wang, C. Schlickriede, Y. Tang, J. Deng, K. Li, J. Yang, M. Holynski, et al., A dielectric metasurface optical chip for the generation of cold atoms, Science Advances 6, eabb6667 (2020).