top of page

Locked
Heading 4
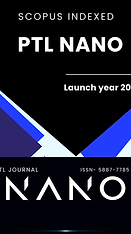

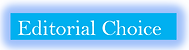
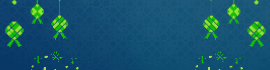

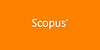
Saturday, July 22, 2023 at 6:30:00 AM UTC
I'm a paragraph. Click here to add your own text and edit me. It's easy.
Rating of Publisher 9
Nanoscience
Heading 6
Heading 6
Superconductivity Induced Ferromagnetism In The Presence of Spin-Orbit Coupling
Yao Lu,1, ∗ I. V. Tokatly,2, 3, 4, †, F. Sebastian Bergeret1, 3, ‡
PTL NANO
Acknowledgements
Y.L. and F.S.B. acknowledge financial support from Spanish AEI through
project PID2020-114252GB-I00 (SPIRIT), TED2021-
130292B-C42, and the Basque Government through
grant IT-1591-22 and IKUR strategy program.
I.V.T. acknowledges support by Grupos Consolidados UPV/EHU del Gobierno Vasco (Grant IT1453-22)
and by the grant PID2020-112811GB-I00 funded by
MCIN/AEI/10.13039/501100011033.
Keyword Highlighted
Superconductivity, Spin-Orbit Coupling, nanotechnology, nanoscience
Unlock Only
Read-only this publication
This option will drive you towards only the selected publication. If you want to save money then choose the full access plan from the right side.
Abstract
We investigate the behavior of magnetic impurities placed on the surface of superconductor thin films with spin-orbit coupling. Our study reveals long-range interactions between the impurities, which decay according to a power law, mediated by the supercurrents. Importantly, these interactions possess a ferromagnetic component when considering the influence of the electromagnetic field, leading to the parallel alignment of the magnetic moments in the case of two impurities. In a Bravais lattice of magnetic impurities, superconductivity facilitates the establishment of ferromagnetic order within specific parameter ranges. These findings challenge the conventional understanding that ferromagnetism and superconductivity are mutually exclusive phenomena. Our theoretical framework provides a plausible explanation for the recently observed remanent flux in iron-based superconductors, particularly Fe(Se,Te).
Introduction
Ferromagnetic ordering is often seen as incompatible with conventional superconductivity due to the presence of an effective exchange field in ferromagnets. This exchange field has the effect of breaking up Cooper pairs, which are composed of electrons in a singlet state [1]. The coexistence of these two orders, however, does exist in hybrid superconductor/ferromagnet (S/F) structures[2, 3]. In such structures, the proximity effect plays a crucial role in enabling the coexistence of superconductivity and ferromagnetism. Singlet pairs can be transformed into triplet pairs through the exchange field of the F region. As a result, a local magnetic moment is produced, extending over distances of the order of superconducting coherence length, ξs. This phenomenon is referred to as the magnetic or inverse proximity effect[4, 5]. This generated magnetic moment is oriented in the opposite direction to the magnetization of the ferromagnetic region. In the case of a small ferromagnetic island, this results in the screening of its magnetic moment[6]. If a second ferromagnetic region (F region) is positioned at a distance smaller than ξs from the first ferromagnet, the energetically favorable arrangement is an antiparallel orientation of the magnetizations of the two F regions. This anti-parallel alignment serves as the basis for the FSF superconducting spin-valve[7–10]. The studies on FSF structures with conventional superconductors indicate an anti-ferromagnetic coupling between the magnets, mediated by the inverse proximity effect. This coupling strength decreases exponentially with the distance between the ferromagnetic regions. This situation changes in thin superconducting films with spin-orbit coupling (SOC). The combination of the exchange field generated by a magnetic impurity, m1 in Fig. 1) , and the SOC results in the spontaneous = generation of anomalous currents through the spin-galvanic
Conclusion
In principle, our predictions regarding a ferromagnetic state mediated by superconductivity can be tested in any superconductor that exhibits a significant Rashba SOC or in a Dirac superconductor, with magnetic impurities. One approach to confirm these predictions is by measuring the local magnetic moment. Notably, a recent experiment [19], provides compelling evidence suggesting the observation of such a ferromagnetic state. Specifically, in the study [19], hysteretic magnetization was observed in Fe(SeTe) with Fe impurities. The magnetization disappears at temperatures above the superconducting critical temperature, indicating that the ferromagnetism is induced by the superconductivity. This observation can be considered as evidence of supercurrentmediated magnetic interaction in the presence of surface Dirac states.
Reference
[1] P. Anderson and H. Suhl, Phys. Rev. 116, 898 (1959).
[2] A. I. Buzdin, Rev. Mod. Phys. 77, 935 (2005).
[3] F. Bergeret, A. F. Volkov, and K. B. Efetov, Rev. Mod. Phys. 77, 1321 (2005).
[4] F. Bergeret, A. F. Volkov, and K. Efetov, Phys. Rev. B 69, 174504 (2004).
[5] J. Xia, V. Shelukhin, M. Karpovski, A. Kapitulnik, and A. Palevski, Phys. Rev. Lett. 102, 087004 (2009).
[6] F. Bergeret, A. F. Volkov, and K. Efetov, Europhysics Letters 66, 111 (2004).
[7] P. De Gennes, Physics Letters 23, 10 (1966).
[8] L. Tagirov, Phys. Rev. Lett. 83, 2058 (1999).
[9] Y. A. Izyumov, Y. N. Proshin, and M. G. Khusainov, Physics-Uspekhi 45, 109 (2002).
[10] R. Ojaj¨arvi, F. Bergeret, M. Silaev, and T. T. Heikkil¨a, Phys. Rev. Lett. 128, 167701 (2022).
[11] V. M. Edelstein, Phys. Rev. Lett. 75, 2004 (1995).
[12] F. Konschelle, I. V. Tokatly, and F. S. Bergeret, Phys. Rev. B 92, 125443 (2015).
[13] F. S. Bergeret and I. V. Tokatly, Phys. Rev. B 102, 060506 (2020).
[14] S. S. Pershoguba, K. Bj¨ornson, A. M. Black-Schaffer, and A. V. Balatsky, Phys. Rev. Lett. 115, 116602 (2015).
[15] A. Mal’Shukov, Phys. Rev. B 98, 054504 (2018).
[16] J. Pearl, Applied Physics Letters 5, 65 (1964).
[17] L. V. Keldysh, JETP Lett. 29, 658 (1979), [Pis’ma Zh. Eksp. Teor. Fiz. 29, 716 (1979)].
[18] P. Cudazzo, I. V. Tokatly, and A. Rubio, Phys. Rev. B 84, 085406 (2011).
[19] B. K. Xiang 1, Y. S. Lin, Q. S. He, J. J. Zhu, B. R. Chen, Y. F. Wang, K. Y. Liang, Z. J. Li, H. X. Yao, M. H. Fang, Y. Lu, F. S. Bergeret, I. V. Tokatly, F. S. Bergeret, Y. H. Wang, ”Observation of long-range ferromagnetism via anomalous supercurrent in a spin-orbit coupled superconductor”.
[20] F. Bergeret and I. Tokatly, Europhysics Letters 110,
57005 (2015).
[21] E. Strambini, A. Iorio, O. Durante, R. Citro, C. SanzFern´andez, C. Guarcello, I. V. Tokatly, A. Braggio,
M. Rocci, N. Ligato, et al., Nature Nanotechnology 15, 656 (2020).
[22] Supplementary material includes the derivations of the simplified free energy, the supercurrent-mediated magnetic interaction, the superfluid weight of a 2D Rashba superconductor and the Γ parameter for a 2D Rashba superconductor.
[23] A. Zyuzin, M. Alidoust, and D. Loss, Phys. Rev. B 93, 214502 (2016).
[24] T. Kokkeler, A. Golubov, and F. Bergeret, Phys. Rev. B 106, 214504 (2022).
[25] Y. Lin, S. Wang, X. Zhang, Y. Feng, Y. Pan, H. Ru, J. Zhu, B. Xiang, K. Liu, C. Zheng, et al., Phys. Rev. X 13, 011046 (2023).
[26] V. Rozenbaum, Zh. Eksp. Teor. Fiz 99, 1836 (1991).
[27] K. Jiang, X. Dai, and Z. Wang, Phys. Rev. X 9, 011033 (2019).
[28] V. Thampy, J. Kang, J. Rodriguez-Rivera, W. Bao, A. T. Savici, J. Hu, T. Liu, B. Qian, D. Fobes, Z. Mao, et al., Phys. Rev. Lett. 108, 107002 (2012).
[29] M. Houzet and J. S. Meyer, Phys. Rev. B 92, 014509 (2015).
bottom of page